Energy Harvesting and Storage Related Research:
The current research is mainly focused on fundamental, transformative and innovative biomaterials, biosensors and energy related research directed at fostering clean energy, regenerative therapies, national security, human welfare and next generation workforce. The main focus of research in all of these areas will be to develop (a) rapid experimental synthesis and processing tools; quantitative analytical and characterization tools; accelerated testing and rapid prototyping; techniques to validate and advance bio materials and materials theory and (b) computational tools for predictive modeling, exploration, simulation and design.
The ultimate vision of energy related research is the development of a coherent computational model and concomitant advanced experimental tools enabling rapid screening, development and manufacturing of advanced energy related materials with significant cost benefits. The research group focuses on identification of ultra-low noble metal/non-noble metal electrocatalysts (EC) and photoelectrocatalyst (PEC) for water electrolysis, fuel cell and air battery, and carbon capture through CO2 conversion to fuel. In the field of electrical energy storage technologies based on rechargeable batteries (Li-ion, Na- ion, Mg- ion batteries), supercapacitor and flow batteries, the research is directed at fulfilling the vision for meeting the EV (electric vehicle) everywhere grand challenge and Renewable Energy Storage goal of DOE. In this direction, the research is focused at rapid synthesis and advanced characterization of next-generation energy related materials in 1D nanotube, nanowire, 2D nono-film, nano-sheet or 3D hierarchical structures consisting of nano-particles or nanocomposites.
High Efficiency Electrocatalyst and Photoelectrocatalyst
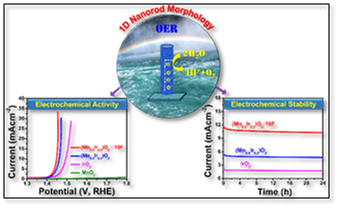
Electrocatalysis (EC) and photoelectrocatalysis (PEC) are the cornerstones of various energy applications including hydrogen evolution/oxidation (HER/HOE), oxygen evolution reaction (OER) (water oxidation), oxygen reduction reaction for hydrogen (ORR), methanol oxidation/reduction, and myriad other chemical processes. In this area, the research group mainly conducts fundamental theoretical and experimental studies to develop cost effective and durable ultra-low platinum group metals (PGM) or PGM-free EC/PEC for OER and ORR. In the present investigation, a predictive design tool and methodology based on theoretical DFT calculation has been developed.
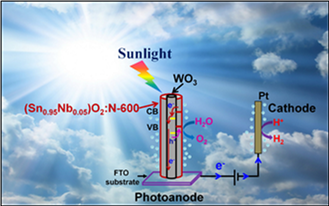
Accordingly, in the experimental section, a 2D thin film model system (e.g. Sn-Ir-oxide, Mn-Ir-oxide OER in PEM based water electrolysis) has been synthesized and characterized for the electronic, atomic/molecular level structure, while also assessing the energy conversion/storage properties to exactly understand the process-structure-property relationships. Such structure-property relationships will be very helpful for the design and synthesis of suitable electro-catalyst in different "material length scale" (e.g. 0D, 1D and 3D nanostructured dimension) as well as serving to ultimately develop PGM-free electro-catalysts. The theoretical and experimental studies will thus work synergistically to determine not only the bulk and surface atomic and electronic level structure and composition that are effective in terms of EC/PEC response but also help to understand the underlying detailed mechanisms. Understanding of the mechanisms will then serve to effectively fine tune and design the EC/PEC system helping to unravel the fundamental pathways leading to the identification of high efficient EC/PEC for various energy storage and conversion.
Rechargeable Battery Research:
Among the different EES technologies developed based on the fundamental electrochemical reaction, lithium batteries (LBs) have emerged as the flagship technologies offering the much desired panacea for high energy and high power applications such as in the advanced portable electronics, electric powered vehicle, military applications as well as stand-alone stationary power systems integrated into the electric grid. Since the commercialization of Li-ion batteries by Sony, lithium ion chemistries represent a significant step forward in battery technology having a high energy density, safety and are ideal for cyclic applications.
Stable operation of Si based anode for Li ion batteries
Silicon (Si) has been extensively studied as high capacity anode for next generation high energy Li-ion batteries (LIBs). However, fast capacity fade still largely limits its practical applications. Due to pulverization and subsequent loss of electrical contact, repeated breaking/formation of solid electrolyte interphase (SEI) and continuous consumption of electrolyte are the major problems caused by the large volume change during lithiation and delithiation. The main goal of this project is to develop low cost synthesis approaches for Si-containing anode materials to enable their practical application in Li-ion batteries (LIB) for plug-in hybrid electric vehicles (PHEV) and electric vehicles (EV). The developed Si based anode has a capacity exceeding 1000 mAh/g while retaining 80% of capacity in EV battery cycles. The research focuses on the development of innovative approaches to generate novel Si/C and Si/lightweight-inactive-matrix (LIM)-based anodes as well as novel morphology of Si-based anodes such as ex situ “core shell” amorphous and nanocrystalline (nc) nanoparticles, hollow nanostructures (nanowires, nanorods, nanotubes, nanoflakes), nanoscale droplets, on myriad C and other LIMs by novel low-cost, viable, HEMM and economical template-derived chemical and solution approaches using economical, environmentally benign, and safe Si precursors to overcome the problems associated with large first cycle irreversible (FIR) capacity loss, while achieving acceptable coulombic efficiencies and cyclability by minimizing the colossal volume expansion related to Si cycling and reversible capacity limitations.
- Rigved Epur, Madhumati Ramanathan, Moni K. Datta, Dae Ho Hong, Prashanth H. Jampani, Bharat Gattu, Prashant N. Kumta, “Scribable multi-walled carbon nanotube-silicon nanocomposites: a viable lithium-ion battery system”, Nanoscale, 2015,7, 3504-3510.
- Rigved Epur, Prashanth H. Jampani, Moni K. Datta, Dae Ho Hong, , Bharat Gattu, Prashant N. Kumta, “A simple and scalable approach to hollow silicon nanotube (h-SiNT) anode architectures of superior electrochemical stability and reversible capacity”, J. Mater. Chem. A, 2015,3, 11117-11129.
- Bharat Gattu, Rigved Epur, Prashanth H. Jampani, Ramalinga Kuruba, Moni K. Datta and Prashant N. Kumta, “Silicon-Carbon Core-Shell (C@Si@C) Hollow Nanotubular Configuration - High Performance Lithium-Ion Anodes”, Journal of Physical Chemistry - C, 2017, 121 (18), pp 9662–9671.
- Bharat Gattu, Prashanth H. Jampani, Moni K. Datta and Prashant N. Kumta, “Water-soluble template derived nanoscale silicon nano-flakes and nano-rods morphologies: stable architectures for lithium ion anodes”, Nanoresearch, 2017.
- B. Gattu, R. Epur, P. Shanti, P.H. Jampani, R. Kuruba, M.K. Datta, A. Manivannan, P.N. Kumta, “Pulsed current electrodeposition of silicon thin film anodes for lithium-ion battery applications”, Inorganics, 2017, 5(2), 27.
Beyond Li-ion batteries:
-sulfur (Li-S) batteries, Li-oxygen (Li-O2) batteries, Li metal anode vs ultra-low cobalt content intercalation type cathode batteries, etc., indicate a huge increase in theoretical energy density relative to the current LIBs (Li-O2, 3505 Wh/kg; Li-S, 2600 Wh/kg). A safe and efficient operation of lithium metal anodes will therefore decide the fate for next-generation energy storage systems of cost £$75/kWh, including rechargeable Li-air batteries, Li-sulfur batteries, and future Li metal batteries.
Engineering Approaches to Dendrite-free Li metal anodes:
³ 400 Wh/kg and ≥750Wh/l with a cost target $75/kWh and cycle life of at least 1,000 cycles for meeting the EV everywhere blueprint.
Electrochemically Stable High Energy Density Lithium-Sulfur Batteries
Lithium–sulfur battery (LSB) technology is widely investigated as an attractive alternative to currently used Li-ion battery (LIB) chemistries for the EV/PEV industry due to the superior theoretical capacity (1674 mAh/g) and specific energy density (2600 Wh/kg) of elemental sulfur. However, the dissolution of sulfur via formation of soluble polysulfides (PSs) (i.e. poor capacity retention) and the inferior electronic conductivity of S (barrier to active materials utilization) are primary limitations of LSB’s continuing to hinder the much awaited commercialization path. Current generation sulfur cathodes show low specific storage capacity, very poor charging rates and low loading densities. To obviate the primary problems of (a) low overall electrode capacity (mAh/g-active material) occurring due to low electronic conductivity of sulfur (b) poor cycling stability owing to polysulfide (PS) dissolution (c) voltage drop due to PS transport across and deposition at lithium anode (d) poor coulombic efficiency (CE), the present work involves identification and synthesis of novel Li ion conductor matrix-coated sulfur nanoparticles and composite sulfur cathodes using complex framework materials, and doped sulfur cathodes consisting of sulfur electrocatalyst for rapid conversion of polysulfides to lithium disulfide. The work involves incorporation of the optimized electrodes into lithium-S pouch cells and evaluation and optimization of electrode properties to maximize capacity, reduce fade and improve coulombic efficiency. Work is also driven towards optimization of electrode characteristics using morphological engineering and 3-D engineering approaches including 3-D printing to improve the interfacial characteristics of the Li-S full-cells.
Beyond Li batteries
The Li-ion arena has witnessed extensive research and is currently the flag ship energy storage system (ESS) for all consumer, portable, and professional applications. Safety and cost are still major hurdles attracting interest in other systems. The ability of Na+ and Mg +2 to cycle similar to Li+ with a theoretical capacity of 1100 Ah/kg combined with its larger abundance and lower costs enables Na and Mg based systems with possible energy densities of ~500 Wh/kg in the 3-5 V open circuit voltage range a feasible option for automotive transportation, grid scale, and defense related energy storage applications. The current work is focused on theoretical and experimental effort to develop revolutionary Na+ rechargeable batteries based energy storage strategies focused on fundamental science, and technology. The current research is focused on identification and development of approaches to generate novel anodes (e.g. Sn, P) and cathodes (NaMn2O4).